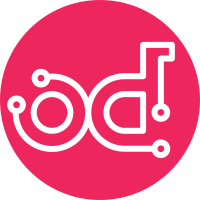
Provides a simple, experimental, CLI tool to generate a composite ring from a list of component builder files. For example: swift-ring-composer <composite-file> compose \ <builder-file> <builder-file> --output <ring-file> Commands available: - compose: compose a list of builder file to a composite ring - show: show the metadata for a composite ring Co-Authored-By: Kota Tsuyuzaki <tsuyuzaki.kota@lab.ntt.co.jp> Co-Authored-By: Matthew Oliver <matt@oliver.net.au> Change-Id: I25a79e71c13af352e19e4358f60545265b51584f
459 lines
24 KiB
ReStructuredText
459 lines
24 KiB
ReStructuredText
=========
|
||
The Rings
|
||
=========
|
||
|
||
The rings determine where data should reside in the cluster. There is a
|
||
separate ring for account databases, container databases, and individual
|
||
object storage policies but each ring works in the same way. These rings are
|
||
externally managed. The server processes themselves do not modify the
|
||
rings; they are instead given new rings modified by other tools.
|
||
|
||
The ring uses a configurable number of bits from the MD5 hash of an item's path
|
||
as a partition index that designates the device(s) on which that item should
|
||
be stored. The number of bits kept from the hash is known as the partition
|
||
power, and 2 to the partition power indicates the partition count. Partitioning
|
||
the full MD5 hash ring allows the cluster components to process resources in
|
||
batches. This ends up either more efficient or at least less complex than
|
||
working with each item separately or the entire cluster all at once.
|
||
|
||
Another configurable value is the replica count, which indicates how many
|
||
devices to assign for each partition in the ring. By having multiple devices
|
||
responsible for each partition, the cluster can recover from drive or network
|
||
failures.
|
||
|
||
Devices are added to the ring to describe the capacity available for
|
||
partition replica assignments. Devices are placed into failure domains
|
||
consisting of region, zone, and server. Regions can be used to describe
|
||
geographical systems characterized by lower bandwidth or higher latency between
|
||
machines in different regions. Many rings will consist of only a single
|
||
region. Zones can be used to group devices based on physical locations, power
|
||
separations, network separations, or any other attribute that would lessen
|
||
multiple replicas being unavailable at the same time.
|
||
|
||
Devices are given a weight which describes the relative storage capacity
|
||
contributed by the device in comparison to other devices.
|
||
|
||
When building a ring, replicas for each partition will be assigned to devices
|
||
according to the devices' weights. Additionally, each replica of a partition
|
||
will preferentially be assigned to a device whose failure domain does not
|
||
already have a replica for that partition. Only a single replica of a
|
||
partition may be assigned to each device - you must have at least as many
|
||
devices as replicas.
|
||
|
||
.. _ring_builder:
|
||
|
||
------------
|
||
Ring Builder
|
||
------------
|
||
|
||
The rings are built and managed manually by a utility called the ring-builder.
|
||
The ring-builder assigns partitions to devices and writes an optimized
|
||
structure to a gzipped, serialized file on disk for shipping out to the
|
||
servers. The server processes check the modification time of the file
|
||
occasionally and reload their in-memory copies of the ring structure as needed.
|
||
Because of how the ring-builder manages changes to the ring, using a slightly
|
||
older ring usually just means that for a subset of the partitions the device
|
||
for one of the replicas will be incorrect, which can be easily worked around.
|
||
|
||
The ring-builder also keeps a separate builder file which includes the ring
|
||
information as well as additional data required to build future rings. It is
|
||
very important to keep multiple backup copies of these builder files. One
|
||
option is to copy the builder files out to every server while copying the ring
|
||
files themselves. Another is to upload the builder files into the cluster
|
||
itself. Complete loss of a builder file will mean creating a new ring from
|
||
scratch, nearly all partitions will end up assigned to different devices, and
|
||
therefore nearly all data stored will have to be replicated to new locations.
|
||
So, recovery from a builder file loss is possible, but data will definitely be
|
||
unreachable for an extended time.
|
||
|
||
-------------------
|
||
Ring Data Structure
|
||
-------------------
|
||
|
||
The ring data structure consists of three top level fields: a list of devices
|
||
in the cluster, a list of lists of device ids indicating partition to device
|
||
assignments, and an integer indicating the number of bits to shift an MD5 hash
|
||
to calculate the partition for the hash.
|
||
|
||
***************
|
||
List of Devices
|
||
***************
|
||
|
||
The list of devices is known internally to the Ring class as ``devs``. Each
|
||
item in the list of devices is a dictionary with the following keys:
|
||
|
||
====== ======= ==============================================================
|
||
id integer The index into the list of devices.
|
||
zone integer The zone in which the device resides.
|
||
region integer The region in which the zone resides.
|
||
weight float The relative weight of the device in comparison to other
|
||
devices. This usually corresponds directly to the amount of
|
||
disk space the device has compared to other devices. For
|
||
instance a device with 1 terabyte of space might have a weight
|
||
of 100.0 and another device with 2 terabytes of space might
|
||
have a weight of 200.0. This weight can also be used to bring
|
||
back into balance a device that has ended up with more or less
|
||
data than desired over time. A good average weight of 100.0
|
||
allows flexibility in lowering the weight later if necessary.
|
||
ip string The IP address or hostname of the server containing the device.
|
||
port int The TCP port on which the server process listens to serve
|
||
requests for the device.
|
||
device string The on-disk name of the device on the server.
|
||
For example: ``sdb1``
|
||
meta string A general-use field for storing additional information for the
|
||
device. This information isn't used directly by the server
|
||
processes, but can be useful in debugging. For example, the
|
||
date and time of installation and hardware manufacturer could
|
||
be stored here.
|
||
====== ======= ==============================================================
|
||
|
||
.. note::
|
||
The list of devices may contain holes, or indexes set to ``None``, for
|
||
devices that have been removed from the cluster. However, device ids are
|
||
reused. Device ids are reused to avoid potentially running out of device id
|
||
slots when there are available slots (from prior removal of devices). A
|
||
consequence of this device id reuse is that the device id (integer value)
|
||
does not necessarily correspond with the chronology of when the device was
|
||
added to the ring. Also, some devices may be temporarily disabled by
|
||
setting their weight to ``0.0``. To obtain a list of active devices (for
|
||
uptime polling, for example) the Python code would look like::
|
||
|
||
devices = list(self._iter_devs())
|
||
|
||
*************************
|
||
Partition Assignment List
|
||
*************************
|
||
|
||
The partition assignment list is known internally to the Ring class as
|
||
``_replica2part2dev_id``. This is a list of ``array('H')``\s, one for each
|
||
replica. Each ``array('H')`` has a length equal to the partition count for the
|
||
ring. Each integer in the ``array('H')`` is an index into the above list of
|
||
devices.
|
||
|
||
So, to create a list of device dictionaries assigned to a partition, the Python
|
||
code would look like::
|
||
|
||
devices = [self.devs[part2dev_id[partition]]
|
||
for part2dev_id in self._replica2part2dev_id]
|
||
|
||
``array('H')`` is used for memory conservation as there may be millions of
|
||
partitions.
|
||
|
||
*********************
|
||
Partition Shift Value
|
||
*********************
|
||
|
||
The partition shift value is known internally to the Ring class as
|
||
``_part_shift``. This value is used to shift an MD5 hash of an item's path to
|
||
calculate the partition on which the data for that item should reside. Only the
|
||
top four bytes of the hash are used in this process. For example, to compute
|
||
the partition for the path ``/account/container/object``, the Python code might
|
||
look like::
|
||
|
||
objhash = md5('/account/container/object').digest()
|
||
partition = struct.unpack_from('>I', objhash)[0] >> self._part_shift
|
||
|
||
For a ring generated with partition power ``P``, the partition shift value is
|
||
``32 - P``.
|
||
|
||
*******************
|
||
Fractional Replicas
|
||
*******************
|
||
|
||
A ring is not restricted to having an integer number of replicas. In order to
|
||
support the gradual changing of replica counts, the ring is able to have a real
|
||
number of replicas.
|
||
|
||
When the number of replicas is not an integer, the last element of
|
||
``_replica2part2dev_id`` will have a length that is less than the partition
|
||
count for the ring. This means that some partitions will have more replicas
|
||
than others. For example, if a ring has ``3.25`` replicas, then 25% of its
|
||
partitions will have four replicas, while the remaining 75% will have just
|
||
three.
|
||
|
||
.. _ring_dispersion:
|
||
|
||
**********
|
||
Dispersion
|
||
**********
|
||
|
||
With each rebalance, the ring builder calculates a dispersion metric. This is
|
||
the percentage of partitions in the ring that have too many replicas within a
|
||
particular failure domain.
|
||
|
||
For example, if you have three servers in a cluster but two replicas for a
|
||
partition get placed onto the same server, that partition will count towards
|
||
the dispersion metric.
|
||
|
||
A lower dispersion value is better, and the value can be used to find the
|
||
proper value for "overload".
|
||
|
||
.. _ring_overload:
|
||
|
||
********
|
||
Overload
|
||
********
|
||
|
||
The ring builder tries to keep replicas as far apart as possible while
|
||
still respecting device weights. When it can't do both, the overload
|
||
factor determines what happens. Each device may take some extra
|
||
fraction of its desired partitions to allow for replica dispersion;
|
||
once that extra fraction is exhausted, replicas will be placed closer
|
||
together than is optimal for durability.
|
||
|
||
Essentially, the overload factor lets the operator trade off replica
|
||
dispersion (durability) against device balance (uniform disk usage).
|
||
|
||
The default overload factor is ``0``, so device weights will be strictly
|
||
followed.
|
||
|
||
With an overload factor of ``0.1``, each device will accept 10% more
|
||
partitions than it otherwise would, but only if needed to maintain
|
||
dispersion.
|
||
|
||
Example: Consider a 3-node cluster of machines with equal-size disks;
|
||
let node A have 12 disks, node B have 12 disks, and node C have only
|
||
11 disks. Let the ring have an overload factor of ``0.1`` (10%).
|
||
|
||
Without the overload, some partitions would end up with replicas only
|
||
on nodes A and B. However, with the overload, every device is willing
|
||
to accept up to 10% more partitions for the sake of dispersion. The
|
||
missing disk in C means there is one disk's worth of partitions that
|
||
would like to spread across the remaining 11 disks, which gives each
|
||
disk in C an extra 9.09% load. Since this is less than the 10%
|
||
overload, there is one replica of each partition on each node.
|
||
|
||
However, this does mean that the disks in node C will have more data
|
||
on them than the disks in nodes A and B. If 80% full is the warning
|
||
threshold for the cluster, node C's disks will reach 80% full while A
|
||
and B's disks are only 72.7% full.
|
||
|
||
-------------------------------
|
||
Partition & Replica Terminology
|
||
-------------------------------
|
||
|
||
All descriptions of consistent hashing describe the process of breaking the
|
||
keyspace up into multiple ranges (vnodes, buckets, etc.) - many more than the
|
||
number of "nodes" to which keys in the keyspace must be assigned. Swift calls
|
||
these ranges `partitions` - they are partitions of the total keyspace.
|
||
|
||
Each partition will have multiple replicas. Every replica of each partition
|
||
must be assigned to a device in the ring. When describing a specific replica
|
||
of a partition (like when it's assigned a device) it is described as a
|
||
`part-replica` in that it is a specific `replica` of the specific `partition`.
|
||
A single device will likely be assigned different replicas from many
|
||
partitions, but it may not be assigned multiple replicas of a single partition.
|
||
|
||
The total number of partitions in a ring is calculated as ``2 **
|
||
<part-power>``. The total number of part-replicas in a ring is calculated as
|
||
``<replica-count> * 2 ** <part-power>``.
|
||
|
||
When considering a device's `weight` it is useful to describe the number of
|
||
part-replicas it would like to be assigned. A single device, regardless of
|
||
weight, will never hold more than ``2 ** <part-power>`` part-replicas because
|
||
it can not have more than one replica of any partition assigned. The number of
|
||
part-replicas a device can take by weights is calculated as its `parts-wanted`.
|
||
The true number of part-replicas assigned to a device can be compared to its
|
||
parts-wanted similarly to a calculation of percentage error - this deviation in
|
||
the observed result from the idealized target is called a device's `balance`.
|
||
|
||
When considering a device's `failure domain` it is useful to describe the number
|
||
of part-replicas it would like to be assigned. The number of part-replicas
|
||
wanted in a failure domain of a tier is the sum of the part-replicas wanted in
|
||
the failure domains of its sub-tier. However, collectively when the total
|
||
number of part-replicas in a failure domain exceeds or is equal to ``2 **
|
||
<part-power>`` it is most obvious that it's no longer sufficient to consider
|
||
only the number of total part-replicas, but rather the fraction of each
|
||
replica's partitions. Consider for example a ring with 3 replicas and 3
|
||
servers: while dispersion requires that each server hold only ⅓ of the total
|
||
part-replicas, placement is additionally constrained to require ``1.0`` replica
|
||
of *each* partition per server. It would not be sufficient to satisfy
|
||
dispersion if two devices on one of the servers each held a replica of a single
|
||
partition, while another server held none. By considering a decimal fraction
|
||
of one replica's worth of partitions in a failure domain we can derive the
|
||
total part-replicas wanted in a failure domain (``1.0 * 2 ** <part-power>``).
|
||
Additionally we infer more about `which` part-replicas must go in the failure
|
||
domain. Consider a ring with three replicas and two zones, each with two
|
||
servers (four servers total). The three replicas worth of partitions will be
|
||
assigned into two failure domains at the zone tier. Each zone must hold more
|
||
than one replica of some partitions. We represent this improper fraction of a
|
||
replica's worth of partitions in decimal form as ``1.5`` (``3.0 / 2``). This
|
||
tells us not only the *number* of total partitions (``1.5 * 2 **
|
||
<part-power>``) but also that *each* partition must have `at least` one replica
|
||
in this failure domain (in fact ``0.5`` of the partitions will have 2
|
||
replicas). Within each zone the two servers will hold ``0.75`` of a replica's
|
||
worth of partitions - this is equal both to "the fraction of a replica's worth
|
||
of partitions assigned to each zone (``1.5``) divided evenly among the number
|
||
of failure domains in its sub-tier (2 servers in each zone, i.e. ``1.5 / 2``)"
|
||
but *also* "the total number of replicas (``3.0``) divided evenly among the
|
||
total number of failure domains in the server tier (2 servers × 2 zones = 4,
|
||
i.e. ``3.0 / 4``)". It is useful to consider that each server in this ring
|
||
will hold only ``0.75`` of a replica's worth of partitions which tells that any
|
||
server should have `at most` one replica of a given partition assigned. In the
|
||
interests of brevity, some variable names will often refer to the concept
|
||
representing the fraction of a replica's worth of partitions in decimal form as
|
||
*replicanths* - this is meant to invoke connotations similar to ordinal numbers
|
||
as applied to fractions, but generalized to a replica instead of a four\*th* or
|
||
a fif\*th*. The "n" was probably thrown in because of Blade Runner.
|
||
|
||
-----------------
|
||
Building the Ring
|
||
-----------------
|
||
|
||
First the ring builder calculates the replicanths wanted at each tier in the
|
||
ring's topology based on weight.
|
||
|
||
Then the ring builder calculates the replicanths wanted at each tier in the
|
||
ring's topology based on dispersion.
|
||
|
||
Then the ring builder calculates the maximum deviation on a single device
|
||
between its weighted replicanths and wanted replicanths.
|
||
|
||
Next we interpolate between the two replicanth values (weighted & wanted) at
|
||
each tier using the specified overload (up to the maximum required overload).
|
||
It's a linear interpolation, similar to solving for a point on a line between
|
||
two points - we calculate the slope across the max required overload and then
|
||
calculate the intersection of the line with the desired overload. This
|
||
becomes the target.
|
||
|
||
From the target we calculate the minimum and maximum number of replicas any
|
||
partition may have in a tier. This becomes the `replica-plan`.
|
||
|
||
Finally, we calculate the number of partitions that should ideally be assigned
|
||
to each device based the replica-plan.
|
||
|
||
On initial balance (i.e., the first time partitions are placed to generate a
|
||
ring) we must assign each replica of each partition to the device that desires
|
||
the most partitions excluding any devices that already have their maximum
|
||
number of replicas of that partition assigned to some parent tier of that
|
||
device's failure domain.
|
||
|
||
When building a new ring based on an old ring, the desired number of
|
||
partitions each device wants is recalculated from the current replica-plan.
|
||
Next the partitions to be reassigned are gathered up. Any removed devices have
|
||
all their assigned partitions unassigned and added to the gathered list. Any
|
||
partition replicas that (due to the addition of new devices) can be spread out
|
||
for better durability are unassigned and added to the gathered list. Any
|
||
devices that have more partitions than they now desire have random partitions
|
||
unassigned from them and added to the gathered list. Lastly, the gathered
|
||
partitions are then reassigned to devices using a similar method as in the
|
||
initial assignment described above.
|
||
|
||
Whenever a partition has a replica reassigned, the time of the reassignment is
|
||
recorded. This is taken into account when gathering partitions to reassign so
|
||
that no partition is moved twice in a configurable amount of time. This
|
||
configurable amount of time is known internally to the RingBuilder class as
|
||
``min_part_hours``. This restriction is ignored for replicas of partitions on
|
||
devices that have been removed, as device removal should only happens on device
|
||
failure and there's no choice but to make a reassignment.
|
||
|
||
The above processes don't always perfectly rebalance a ring due to the random
|
||
nature of gathering partitions for reassignment. To help reach a more balanced
|
||
ring, the rebalance process is repeated a fixed number of times until the
|
||
replica-plan is fulfilled or unable to be fulfilled (indicating we probably
|
||
can't get perfect balance due to too many partitions recently moved).
|
||
|
||
|
||
.. _composite_rings:
|
||
|
||
---------------
|
||
Composite Rings
|
||
---------------
|
||
.. automodule:: swift.common.ring.composite_builder
|
||
|
||
**********************************
|
||
swift-ring-composer (Experimental)
|
||
**********************************
|
||
.. automodule:: swift.cli.ringcomposer
|
||
|
||
---------------------
|
||
Ring Builder Analyzer
|
||
---------------------
|
||
.. automodule:: swift.cli.ring_builder_analyzer
|
||
|
||
-------
|
||
History
|
||
-------
|
||
|
||
The ring code went through many iterations before arriving at what it is now
|
||
and while it has largely been stable, the algorithm has seen a few tweaks or
|
||
perhaps even fundamentally changed as new ideas emerge. This section will try
|
||
to describe the previous ideas attempted and attempt to explain why they were
|
||
discarded.
|
||
|
||
A "live ring" option was considered where each server could maintain its own
|
||
copy of the ring and the servers would use a gossip protocol to communicate the
|
||
changes they made. This was discarded as too complex and error prone to code
|
||
correctly in the project timespan available. One bug could easily gossip bad
|
||
data out to the entire cluster and be difficult to recover from. Having an
|
||
externally managed ring simplifies the process, allows full validation of data
|
||
before it's shipped out to the servers, and guarantees each server is using a
|
||
ring from the same timeline. It also means that the servers themselves aren't
|
||
spending a lot of resources maintaining rings.
|
||
|
||
A couple of "ring server" options were considered. One was where all ring
|
||
lookups would be done by calling a service on a separate server or set of
|
||
servers, but this was discarded due to the latency involved. Another was much
|
||
like the current process but where servers could submit change requests to the
|
||
ring server to have a new ring built and shipped back out to the servers. This
|
||
was discarded due to project time constraints and because ring changes are
|
||
currently infrequent enough that manual control was sufficient. However, lack
|
||
of quick automatic ring changes did mean that other components of the system
|
||
had to be coded to handle devices being unavailable for a period of hours until
|
||
someone could manually update the ring.
|
||
|
||
The current ring process has each replica of a partition independently assigned
|
||
to a device. A version of the ring that used a third of the memory was tried,
|
||
where the first replica of a partition was directly assigned and the other two
|
||
were determined by "walking" the ring until finding additional devices in other
|
||
zones. This was discarded due to the loss of control over how many replicas for
|
||
a given partition moved at once. Keeping each replica independent allows for
|
||
moving only one partition replica within a given time window (except due to
|
||
device failures). Using the additional memory was deemed a good trade-off for
|
||
moving data around the cluster much less often.
|
||
|
||
Another ring design was tried where the partition to device assignments weren't
|
||
stored in a big list in memory but instead each device was assigned a set of
|
||
hashes, or anchors. The partition would be determined from the data item's hash
|
||
and the nearest device anchors would determine where the replicas should be
|
||
stored. However, to get reasonable distribution of data each device had to have
|
||
a lot of anchors and walking through those anchors to find replicas started to
|
||
add up. In the end, the memory savings wasn't that great and more processing
|
||
power was used, so the idea was discarded.
|
||
|
||
A completely non-partitioned ring was also tried but discarded as the
|
||
partitioning helps many other components of the system, especially replication.
|
||
Replication can be attempted and retried in a partition batch with the other
|
||
replicas rather than each data item independently attempted and retried. Hashes
|
||
of directory structures can be calculated and compared with other replicas to
|
||
reduce directory walking and network traffic.
|
||
|
||
Partitioning and independently assigning partition replicas also allowed for
|
||
the best-balanced cluster. The best of the other strategies tended to give
|
||
±10% variance on device balance with devices of equal weight and ±15% with
|
||
devices of varying weights. The current strategy allows us to get ±3% and ±8%
|
||
respectively.
|
||
|
||
Various hashing algorithms were tried. SHA offers better security, but the ring
|
||
doesn't need to be cryptographically secure and SHA is slower. Murmur was much
|
||
faster, but MD5 was built-in and hash computation is a small percentage of the
|
||
overall request handling time. In all, once it was decided the servers wouldn't
|
||
be maintaining the rings themselves anyway and only doing hash lookups, MD5 was
|
||
chosen for its general availability, good distribution, and adequate speed.
|
||
|
||
The placement algorithm has seen a number of behavioral changes for
|
||
unbalanceable rings. The ring builder wants to keep replicas as far apart as
|
||
possible while still respecting device weights. In most cases, the ring
|
||
builder can achieve both, but sometimes they conflict. At first, the behavior
|
||
was to keep the replicas far apart and ignore device weight, but that made it
|
||
impossible to gradually go from one region to two, or from two to three. Then
|
||
it was changed to favor device weight over dispersion, but that wasn't so good
|
||
for rings that were close to balanceable, like 3 machines with 60TB, 60TB, and
|
||
57TB of disk space; operators were expecting one replica per machine, but
|
||
didn't always get it. After that, overload was added to the ring builder so
|
||
that operators could choose a balance between dispersion and device weights.
|
||
In time the overload concept was improved and made more accurate.
|
||
|
||
For more background on consistent hashing rings, please see
|
||
:doc:`ring_background`.
|